Hydropower and seasonal electricity storage
A post on why hydropower plants with large reservoirs will be increasingly helpful
The discourse on storage has become a focal point in the energy landscape, and this trajectory is undoubtedly poised to persist. When delving into storage within the power sector, the conversation often revolves around additional components like batteries, flywheels, hydrogen, and the like. Nevertheless, we have been around an already-stored renewable energy: hydropower with large reservoirs1.
How much reserve do we have? Where is it located? Why does it matter?
I will try to answer these questions here, so let’s dig in together.
In this post, I will use some elements from the book Monetizing Energy Storage. This is a great reference that I recommend for all persons interested in storage.
The different kinds of storage
The storage of energy, specifically electricity, can be categorized in diverse ways, contingent upon the specific service that the storage is intended to deliver. The clusterification can be organized based on the duration requirements, as illustrated below. In this context, duration refers to the period during which the energy needs to be stored before release. Consequently, four distinct groups can be identified:
Group 1: Long-duration storage (weeks to months), or seasonal storage. For example, storing excess solar energy in summer and releasing it in winter. Or storing the excess hydro of spring and releasing it for late summer and early fall2.
Group 2: Medium-long duration (days or day/night). This is principally to perform energy arbitrage, such as managing the daily excess of solar, for example.
Group 3: Short to medium-duration (a few hours maximum) for power reserves. This is mainly for power reserves and ancillary services.
Group 4: Short-duration (a few minutes) storage for power quality mainly.
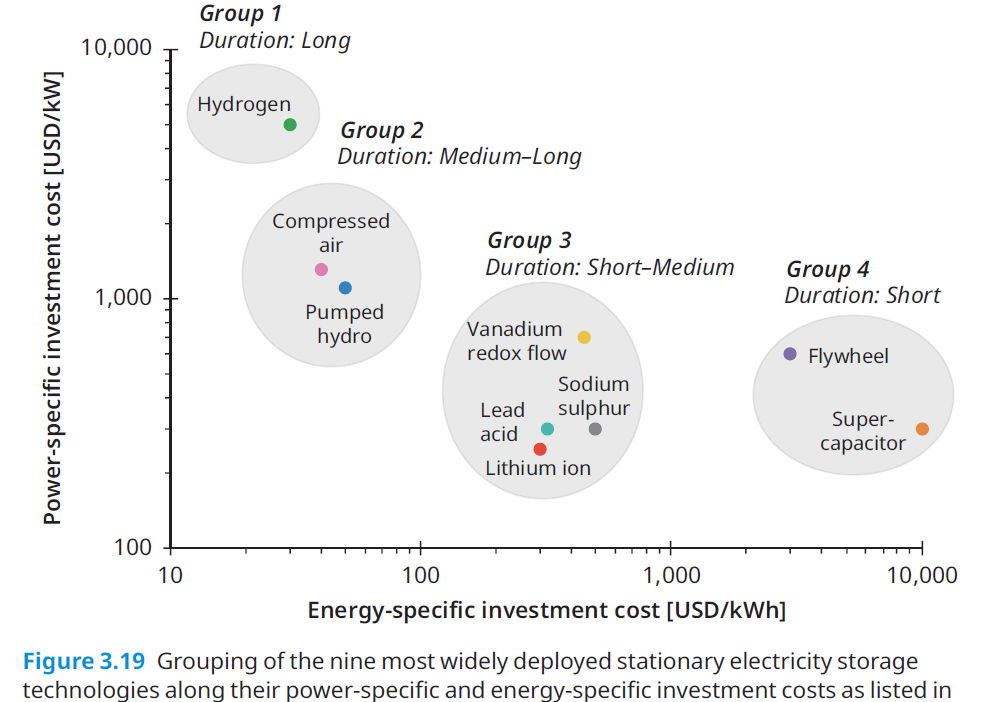
Certainly, these groups are not entirely isolated. Notably, lithium-ion batteries, typically classified in group 3, are gaining prominence in the realm of group 2 activities, particularly in the context of energy arbitrage.
Natural gas as a seasonal storage
Long-duration or seasonal storage involves the capacity to store energy over extended periods, typically spanning several weeks or even months. In both Europe and the United States, the energy demand tends to be significantly higher during winter, primarily driven by heating. To address this seasonal fluctuation, extensive natural gas storage facilities have been developed, with capacities reaching up to one thousand TWh3, acting as a crucial buffer to bridge the gap between winter and summer energy needs.
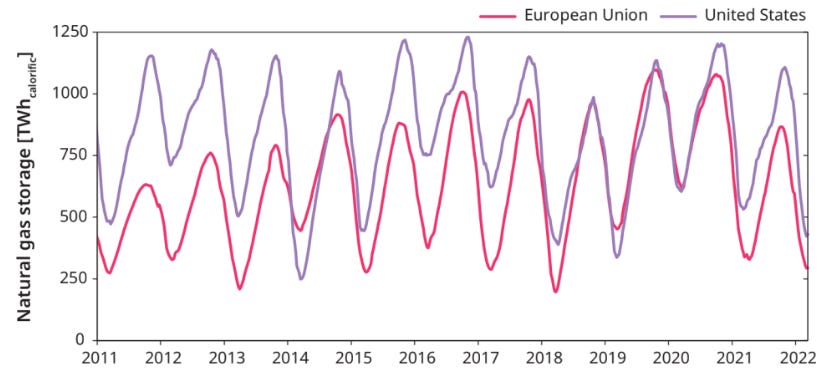
What is the solution without fossil fuel?
From the book mentioned earlier:
These cursory analyses of the required energy storage capacities to decarbonize the electricity and overall energy sectors, reveal the TWh-challenge of converting existing fossil fuel or deploying new storage systems. However, the economic case for these long-duration storage systems may be the hardest challenge to overcome. Chapter 5 shows lifetime cost of >500 USD/MWh (electric) for an exemplary seasonal electricity storage system by 2030-40. The European gas network discharges up to 800 TWh over winter, which equates to approximately 320 TWh of electricity.
I agree with the perspective that one of the most challenging issues in achieving deep decarbonization, particularly in the power sector and across the entire energy landscape, is the imperative for seasonal storage and its associated costs.
The authors advocate for hydrogen as the sole economical solution, albeit acknowledging its considerable expense—exceeding $500 per MWh, approximately 10 times the historical electricity price. This financial hurdle underscores their assertion that this predicament ranks among the most challenging obstacles to overcome in the pursuit of a decarbonized economy.
Nevertheless, other authors suggest lower prices ranging from 207€/MWh to 284€/MWh. Despite the diminished costs, the economic impracticality of storing energy at a scale comparable to existing gas reserves, which often amount to hundreds of TWh, remains evident.
Do we actually need seasonal storage in a decarbonized world?
As we progress toward a decarbonized world, a pertinent question arises: do we truly require the same magnitude of seasonal storage? This query is currently under discussion within the scientific community, with diverse perspectives ranging from conservative estimates of a few days' worth of consumption to more expansive proposals encompassing several months of energy consumption. The reality likely lies somewhere in between these extremes, necessitating a delicate trade-off between potentially overbuilding renewables—with the consequential curtailment of some generation—and investing in storage solutions.
It is important to note that seasonal storage should not only consider the Dunkelflaute4 period but several years as these authors stated:
“While our time series analysis supports previous findings that periods with persistently scarce supply last no longer than two weeks, we find that the maximum energy deficit occurs over a much longer period of nine weeks. This is because multiple scarce periods can closely follow each other.”
Certainly, the requirement for seasonal storage is highly contingent on the unique circumstances of each country, and various factors complicate any broad generalization. Consequently, I refrain from presenting specific numerical figures. However, I lean towards the perspective that we will likely necessitate a buffer encompassing several weeks equivalent to our energy consumption. As a result, our storage requirements could well exceed 100 TWh5, and quite possibly be well above this estimate in my view. While hydrogen could potentially fulfill this need, it comes with a considerable economic cost.
Despite this, it's noteworthy that we sometimes overlook an existing technology that already offers substantial seasonal storage capabilities for the electricity sector: hydropower with large reservoirs.
Can we consider hydro with reservoirs as storage?
Some people may argue that hydro with large reservoirs is not a proper storage facility as we do not store the instantaneous energy surplus from renewables like it is supposedly intended with hydrogen. Nevertheless, I argue two elements:
Firstly, the flow of water in these reservoirs represents a flow of energy. By opting for renewables over water release, we essentially preserve this energy for later use, constituting a form of effective storage in itself.
Secondly, addressing daily surpluses, particularly those stemming from solar energy, can be efficiently managed through energy arbitrage, most probably via a combination of batteries, pumped hydro6, and thermal storage.
Examining the stored energy patterns reveals a discernible seasonal fluctuation, with the lowest levels typically occurring after the heating season—reminiscent of the behavior observed in natural gas storage. Hereunder is for Sweden (up to 30 TWh, or 30,000,000 MWh).
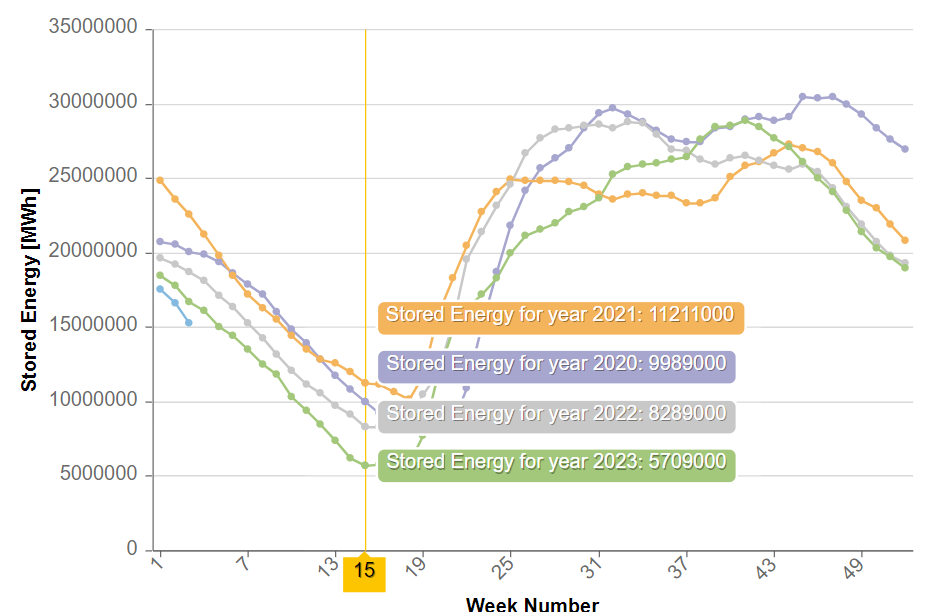
The following graph shows the energy stored for KESH, the public producer in Albania. The highest point is 1,400 GWh or 1.4 TWh. The maximal value is attained in June generally as the snow has completely melted and consumption is picking up due to the need for cooling. The lowest level is generally achieved in October or November when rain is more abundant7.
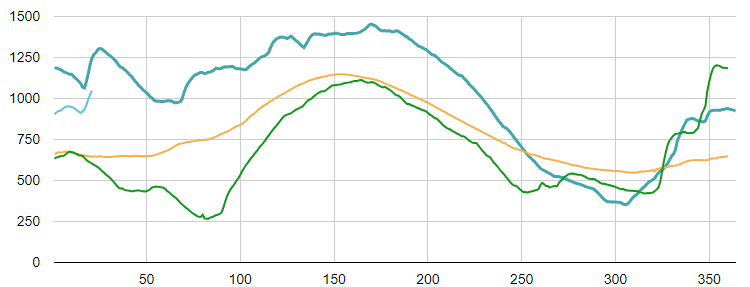
How much hydro reserve do we have in Europe?
The magnitude of this sort of storage varies greatly from one country to another. There are about 130 TWh8 of available storage9 in Europe with a very unequal distribution as we can see from the graph below. It is clear that Norway and to a lesser extent Sweden dominate the share of energy storage. This ability to store large amounts of energy with hydropower is probably a major reason why the share of electricity consumption in the total energy consumption10 is much higher in Nordic countries than in Germany for example.
This recent paper states the following:
When considering the Europe as a whole, it is estimated that European water (not only hydropower) reservoirs provide a theoretical storage capacity of 220 TWh. Norway has the largest (85 TWh) storage capacity in Europe, more than any EU member states, e.g. Austria (3.2 TWh in water reservoirs), France (9.8 TWh), Germany (0.3 TWh), Greece (2.4 TWh), Italy (7.9 TWh), Portugal (2.6 TWh), Spain (18.4 TWh), Sweden (34 TWh) and Finland (5 TWh). Switzerland hosts 8.4 TWh. Norwegian hydropower acts like a battery for balancing variable renewables in neighbouring countries, with more than 1000 reservoirs.
The graph below compares the stored electricity to the overall electricity consumption. It should be noted that the data, except for Albania (which leads to an underestimation11), are from the Entso-e transparency platform concerning stored energy and from various sources concerning electricity consumption12.
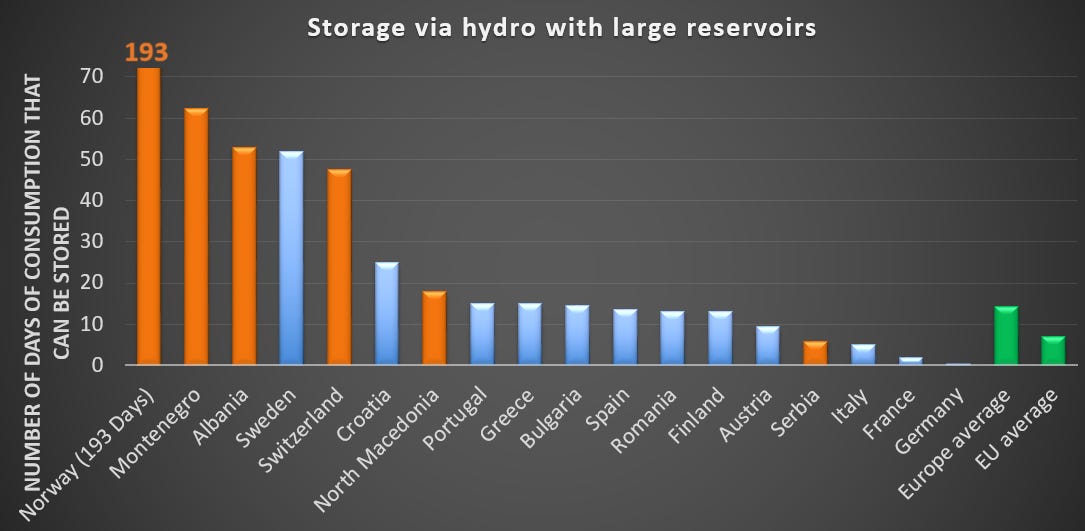
Notably, countries outside the European Union (EU) exhibit a more substantial proportion of stored energy in hydropower plants compared to their EU counterparts. The average duration for Europe, as a collective entity, stands at approximately 14 days, while within the EU alone, it diminishes to 7 days. However, these averages should be regarded as highly theoretical due to constraints imposed by the grid, making it impractical to treat Norway as an oversized European battery.
Furthermore, hydroelectric systems featuring extensive reservoirs encounter various limitations in their utilization. Regulatory constraints, such as those governing water flow management for agricultural purposes or safeguarding marine life, can influence generation patterns.
The increasing value of storage hydropower plants
The transition to a decarbonized economy necessitates a heightened reliance on electricity for both heating and transportation, accompanied by an increased dependency on variable energy sources like solar and wind. As the shift away from fossil fuels progresses, the inherent buffer provided by fossil fuels will inevitably diminish.13
In essence, these hydropower plants are likely to be employed strategically, activated primarily when electricity prices reach levels that justify generation—such as during peak hours and the winter period. As the energy system undergoes the paradigm shift, volatility will undoubtedly increase, rendering electricity buffers such as hydro with large reservoirs increasingly valuable over time. Evidently, hydro can only be used in countries where the potential exists and it means that these countries have an advantage compared to countries that will have to use the much more costly solution of hydrogen as a long-term storage solution.
Hydropower can either be run-of-river or with a reservoir. Run-of-river power plants have little to no stored energy and therefore flexibility for generating power. In addition, there are also pumped hydropower plants where water can be pumped back to a higher reservoir.
This is currently what is being done in Albania, where solar is not yet developed to a large extent.
In calorific terms. As electricity is more efficient for heating, a similar electricity store would be much less in terms of TWh.
Dunkelflaute is a German term that can be translated to "dark doldrums" or "dark lull" in English. It is often used to refer to a period when there is little to no sunlight or wind for generating solar or wind power
Electrical consumption was 2809 TWh in 2022 in the EU. According to Fit-for-55, the electricity consumption would be 3600 TWh in 2030. By considering 2 weeks, this is the equivalent of 138 TWh.
Pumped hydro is the process of pumping water from a lower reservoir to a higher reservoir. Some pumped hydro are situated at the same location as large hydro with reservoirs.
In 2022, due to the energy crisis, there has also been a low level in March.
TWh is one million MWh. 130 TWh is slightly above the total electricity consumption of the Netherlands. For comparison, a Tesla Megapack (container full of batteries) has a storage capacity of 3 MWh.
This figure was calculated by taking the difference between the minimal and maximal levels from 2015 to 2023 of all the countries mentioned on this website.
You can search here for Total Final Consumption (TFC) by source in the topic Energy Consumption to observe the much higher share of electricity in Norway and Sweden compared to countries like Germany.
Please note that the objective is more to illustrate the total storage than to be accurate.
Some might argue that we could aim for an objective with natural gas that can still play the pivotal role of providing the required power for the 5 to 15% time when renewables are insufficient.
Dams have huge environmental impacts: flooding large areas of valuable land, blocking sediment flows and fish migrations, water losses by evaporation and possible catastrophic failure. So we are removing dams, rather than building more of them.
https://www.americanrivers.org/2023/02/dam-removals-continue-across-the-u-s-in-2022/
https://youtu.be/laTIbNVDQN8?si=fdNjasywGTmA0Ap6
https://en.wikipedia.org/wiki/1975_Banqiao_Dam_failure
Interesting article. I like how you differentiate between duration of storage for each technology. I have not seen that before, but it makes a lot of sense.
One additional point. It is not just storing the electricity in hydro-electric dams, it also transporting the electricity to the dams and then back to the consumer at a later date. Given that the vast majority of hydro storage is in Norway and Sweden on the northwest edge of Europe, the geography and distance add another layer of complexity.
For example, can Ireland even transport its excess electricity from wind to Norway or Sweden?