Limits to growth: solar edition
Solar will play a key role in our energy systems but economics will limit its growth
Solar power is undeniably poised for a promising future; a consensus that few would dispute. The recent surge in solar adoption has broken historical records, leading some experts to envision the dawn of a solar-powered world. But are there limits to this bright future?
In this post, we will present what represents for us the most important limiting factor: the decreasing value of additional solar.
The exponential phase
The present progression of solar energy is truly spectacular. In 2022, the world celebrated a remarkable achievement as the first terawatt (TW) of solar capacity was installed globally. Nevertheless, it's vital to acknowledge that this accomplishment constitutes only a relatively small fraction of total electricity production and an even smaller proportion of primary energy consumption1.
Can we expect the continuation of this impressive exponential growth?
Excessively optimistic scenario
Some authors express exceptionally optimistic views about the future. Below is an example that, in our opinion, illustrates an excessive degree of optimism.
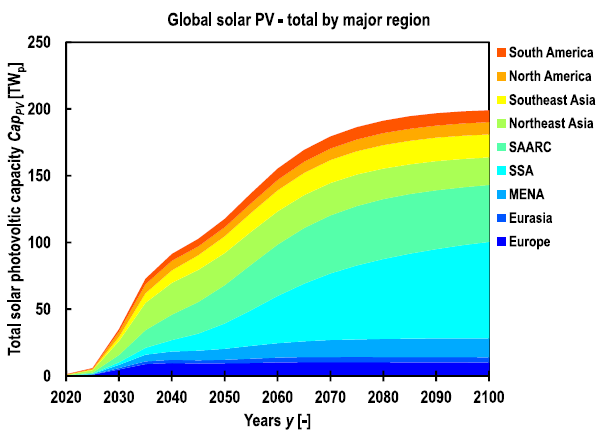
It should be noted that 70 TW is about 6 times more generation capacity than the current global capacity of all technologies combined, which looks extremely challenging to attain from a grid integration perspective at least.
So, no, it's unlikely that we will achieve 70 terawatts (TW) of installed capacity within a mere 12 years. What the eventual figure will be remains uncertain. Predicting long-term trends has proven challenging for human society, and this holds true for forecasting solar capacity, as demonstrated by the fluctuating trend depicted in the graph below.
As a result, we choose not to engage in precise projections, but rather, we opt to emphasize the diminishing economic viability of solar energy, which, in our view, constitutes the most significant constraint.
Spatial and material constraints
But first, let’s explore two constraints that are often cited as limiting factors. The first is the spatial constraint. If we assume that 75 MW can be placed per square kilometer, then achieving one terawatt (TW) would necessitate approximately 13,333 square kilometers of land, equivalent to just 43% of Belgium's land area or a mere 0.009% of the global land area. Except for densely populated regions, it appears that spatial constraints may not be the most significant limitation. While some projects may contend with competing land uses, we assert that the spatial constraint is not the most critical limiting factor2.
Secondly, the question of whether we have a sufficient supply of raw materials is of considerable complexity. Nevertheless, we lack the necessary expertise to provide an exact assessment of this issue. There are conflicting reports on this matter, although we tend to be optimistic. However, it's worth noting that meeting future demand may necessitate increased mining activities, which, in turn, can have social and environmental repercussions.
Hence, we are of the opinion that the primary limitations to substantial expansion are not the spatial and material constraints, but rather the diminishing economic viability. Let's delve into this aspect.
The concept of capture price
Some commentators are prompt to draw comparisons between solar energy and other technologies, particularly in terms of the Levelized Cost of Energy (LCOE)3 . It's evident that in many countries, solar has emerged as the most cost-effective energy source according to this metric.
Regrettably, as we emphasized in a prior post, while LCOE serves as a useful tool for comparing similar technologies, it falls short of providing a comprehensive understanding of total system costs, largely due to its omission of the value generated by the electricity produced. In fact, as solar power becomes increasingly prevalent, market prices tend to decrease, thereby diminishing the additional value of solar.
This decline in market value can be illustrated by the diminishing capture price of solar. The capture price, defined as the weighted average of market prices attributed to solar generation, reflects the price that a solar plant would receive if it were to sell its electricity on the day-ahead exchange. For a simple model, you can read my previous post here.
We are not alone in forecasting some rapid declines in capture prices. For example, S&P Global reports that “solar PV, annual capture rates will fall from 90% harvested in 2022, 80% so far in 2023 to below 20% after 2026, rising above 25% only after 2040.”
Furthermore, this study highlights this remarkable trend. We will not delve into the details but simply, in the current context, an increasing share of variable renewables (VRE) would mean a drastic drop in market value. The share must only be around 18% (55% divided by three4) for the market value of solar (yellow line of the graph on the left) to drop close to zero.
A race between LCOE and capture rates
This diminishing value is expected to outpace the continuous reduction in project costs, particularly in countries where solar adoption is already high5. Remarkably, the cost decrease of solar modules has been so significant that they no longer dominate project expenses in general.
In the case of residential solar installations, personal quotes received in Belgium typically fall within a price range of 1.5-1.7€/Wp, whereas costs tend to be considerably higher in the United States6. Even without factoring in module costs, it is challenging to envision small-scale projects being completed for less than 1 €/Wp installed. With the proliferation of intermittent energy sources, the adoption of dynamic pricing, and the phasing out of net metering, it becomes increasingly likely that surplus energy generation injected into the grid will be compensated at market rates, except for government subsidies (see subsequent section). Obtaining market value for solar energy at the current cost of rooftop solar projects already presents financial challenges in most countries.
As for utility-scale solar projects, while project costs may continue to decrease, our analysis, as outlined in a previous post, indicates that even if module costs were negligible, the LCOE for large solar installations would only decrease by a third over the next decade. This is because module costs are no longer the predominant cost factor. Based on our assessment, it is likely that capture rates will decline at a faster rate, thereby making solar projects less appealing, especially in the current context of high-interest rates.
Naturally, there are significant unknown variables that could play a pivotal role in sustaining the ongoing economic appeal of solar energy.
Unknown 1: Storage
The first significant unknown is our ability to efficiently store substantial quantities of solar energy at a reasonable cost. While storage enhances the capture price, it simultaneously adds to the overall project cost. It is highly likely that, at some point, incorporating storage to shift peak load to the evening hours will make economic sense. However, pinpointing precise figures remains challenging, contingent upon a multitude of cost parameters and potential capture price variations.
Therefore, storage can indeed offer added flexibility but it would be prudent not to anticipate more than a 2 to 4-hour shift in peak load generation, allowing for some additional margin of expansion without fundamentally altering the assessment that capture prices might go well below LCOE of solar projects.
Unknown 2: demand flexibility
The second major unknown revolves around demand flexibility, which involves the ability to align our consumption patterns with solar generation patterns. One prominent contender in this regard is electric mobility, as previously outlined. Another avenue is the integration of specific loads, such as hydrogen electrolyzers, to harness any surplus solar energy. While demand flexibility holds significant promise, substantial practical experience in this domain is currently lacking.
In theory, demand flexibility appears feasible, but its practical and economic viability remains less clear. Do the financial incentives justify altering consumption patterns? It is likely that some relatively minor adjustments, enabled by automation, can be implemented, but achieving large-scale demand flexibility is likely to be considerably more intricate. This is true for households as well as large industries requiring a stable supply of electricity. Consumers are consuming electricity for doing something else while generators are there to produce only. Therefore, it seems natural that consumers, small or large, are bound by more constraints than generators.
Furthermore, the realm of hydrogen production remains in its early stages, and it is probable that peak solar generation can only supply power for a limited number of hours, thus limiting its overall impact. Additionally, the production of green hydrogen relies on access to cost-effective power sources, which may not significantly enhance solar capture rates.
Counteracting this trend: government subsidies
Another pivotal uncertainty lies in the willingness of governments to promote solar expansion, even if it necessitates offering subsidies. There can be compelling reasons to advocate for further solar adoption, including the reduction of carbon emissions and bolstering energy security.
Subsidies can take various forms:
Net Metering: An explicit approach that makes rooftop solar highly economically appealing, as customers can utilize the grid effectively as a large-scale battery and offset grid fees.
Feed-in Tariffs (FIT) or Contract-for-Differences (CfD): These mechanisms ensure that producers receive a predetermined price for their energy, irrespective of market fluctuations.
Fixed Market Premiums: An arrangement where generators receive compensation or tradable certificates for every megawatt-hour (MWh) produced, constituting a fixed or nearly fixed price add-on.
It remains to be seen how committed governments will be to sustaining subsidies, especially if the capture value falls significantly below LCOE.
What is the limit then?
It is difficult to establish a strict limit and probably it does not make much sense, as there are numerous variables, including advancements in solar technology, battery storage, demand flexibility, and government commitment to sustaining growth through subsidies.
Let's assume that the point of significant declining market value begins to emerge at around a 15% penetration rate (see our previous post on the market model). Attaining double this rate, or a global share of 30% of electricity generation, would already be a significant achievement. With the current electricity consumption levels, this equates to 7 TW of installed solar capacity. By 2050, global electricity demand is projected to double to 50,000 TWh, with 30% of that amounting to 15,000 TWh. This translates to approximately 14 TW of installed solar capacity, which is 13 TW more than the current levels. This would require an installation rate of around 500 GW per year, for the next 26 years.
Should we succeed in reaching this milestone, it would already constitute a remarkable success.
Of course, there are way too many unknowns…we won’t probably take the bet ;-)
You can find a shorter version of this post on X/Twitter.
1 TW would generate around 1,200 TWh globally, compared to the 25,500 TWh of electricity consumed in 2022 and 160,000 TWh of primary energy consumed. Of course, by electrifying uses, primary energy consumption will be reduced due to the higher efficiency of electricity.
Especially considering agrivoltaics and vertical solar.
The study assumes an equal share between solar, onshore wind, and offshore wind.
A high share of solar depends on the country. We believe that when the peak solar generation is similar to the peak load, we can start to assume that solar penetration is high, even though it would not represent more than 10 to 20% of the electricity generation.
Around 3 $/W according to this source.
One other constraint to consider is that solar panels real world service life is 15 years before removal and a trip to the dump, notwithstanding that they are an intermittent and diffuse energy generator.
"In fact, as solar power becomes increasingly prevalent, market prices tend to decrease, thereby diminishing the additional value of solar."
Unless I'm missing something, isn't this argument kind of weak? It seems a bit like saying 'as water becomes more available, prices tend to decrease, thereby diminishing the value of additional water infrastructure'. The point isn't that it's valuable, but that citizens have their water and energy needs taken care of.
Practical arguments when it comes to the implementation of renewables, like the disconnect between peak production and peak consumption, the problem of storage and viability of hydrogen infrastructure I find much more convincing.