Aside from the rise of solar energy1, another significant trend currently unfolding is the proliferation of large-scale batteries worldwide, and in Europe in particular. This trend is poised to have a substantial impact on our grids and the electricity markets.
Reading the news, I have been surprised by the numerous new projects and record-setting announcements:
January 2024: The Largest Electric Battery Project in Continental Europe
April 2024: Europe’s largest battery storage project secures approval
October 2024: Estonia begins construction on Europe’s largest battery park
October 2024: Large-scale battery storage in Germany set to increase five-fold within 2 years
November 2024: Central & Eastern Europe: Utility-scale storage market set to increase fivefold by 2030
December 2024: Europe battery capacity to rise 5-fold by 2030 – consultancy
It's not just projections; the growth of large-scale batteries this year has been unprecedented. As the graph below illustrates, European storage capacity is expected to increase from 17.2 GWh to 22.4 GWh. A significant part of this growth is attributed to utility-scale storage, which is projected to rise from 3.6 GWh to 11 GWh—representing a threefold increase. To put it plainly, a 200% growth rate is exceptional.
Such a growth can be seen in all the projects being commercialized recently, here are a few examples: Aghada, Bollingstedt, or Ville-sur-Haine.
Of course, a common critique is that batteries only store currently a few minutes' worth of the total electric load, which is true. As I have described in a previous post, the only truly large-scale storage currently available (capable of postponing weeks of electric consumption) is large-scale hydro with reservoirs, with energy reserves expressed in TWh.
Nevertheless, various aspects of grid operations depend on much shorter-term variations, including all power reserves (such as FCR, aFRR, and mFRR in Europe) and intraday markets. These processes do not require storage for several days; even storage measured in hours would have a significant impact.
Let’s explore further.
Like solar: getting cheaper…
Large-scale batteries share at least two characteristics with solar: it is getting cheap and it is modular. The cost decrease has been phenomenal, as we can observe in the graph below, with a logarithmic Y-scale.
Similarly to solar, where China is largely dominating the whole supply chain, prices are being pushed down further. A BESS2 with a cost below 100 $/kWh, would mean that a 2-hours battery costs only 200 k$/MW.
Of course, this level of project cost in Europe is not (yet) achieved. From the Tesla tool, 20 Megapacks would cost 18.8 M$ for 77 MWh, or 244 $ per kWh. Furthermore, for this particular project3, according to their website, taking a project cost of 250 M€ for 800 MWh, we have a 312 € per kWh. We are therefore still relatively far from reaching below 100 $/kWh in most projects but still, 300 €/kWh means 600 €/kW for a 2-hours battery, way below the cost of any gas peaker plant4.
With the prospects of ever cheaper batteries, it is very likely that an increasing amount of them will be installed, including to replace some of the most expensive gas peaker plants5.
… and modular
In addition to getting cheaper, batteries are extremely modular, something that is very similar with solar, and to a lesser extent wind projects. Large-scale batteries look strangely close to a myriad of lego blocks put side by side over a flat field. From the book Review How Big Things Get Done:
Modularity delivers faster, cheaper, and better, making it valuable for all project types and sizes. The core of modularity is repetition. Put down one Lego block. Snap on another. And another. Repeat, repeat, repeat. Click, click, click. Repetition enables experimentation. If something works, you keep it in the plan. If it doesn’t, you “fail fast” and adjust the plan. Repetition also generates experience, making your performance better (positive learning curve). When you can build modules and deliver them to the site, the building isn’t constructed; it’s assembled, like Lego. Modularity radically reduces risk.
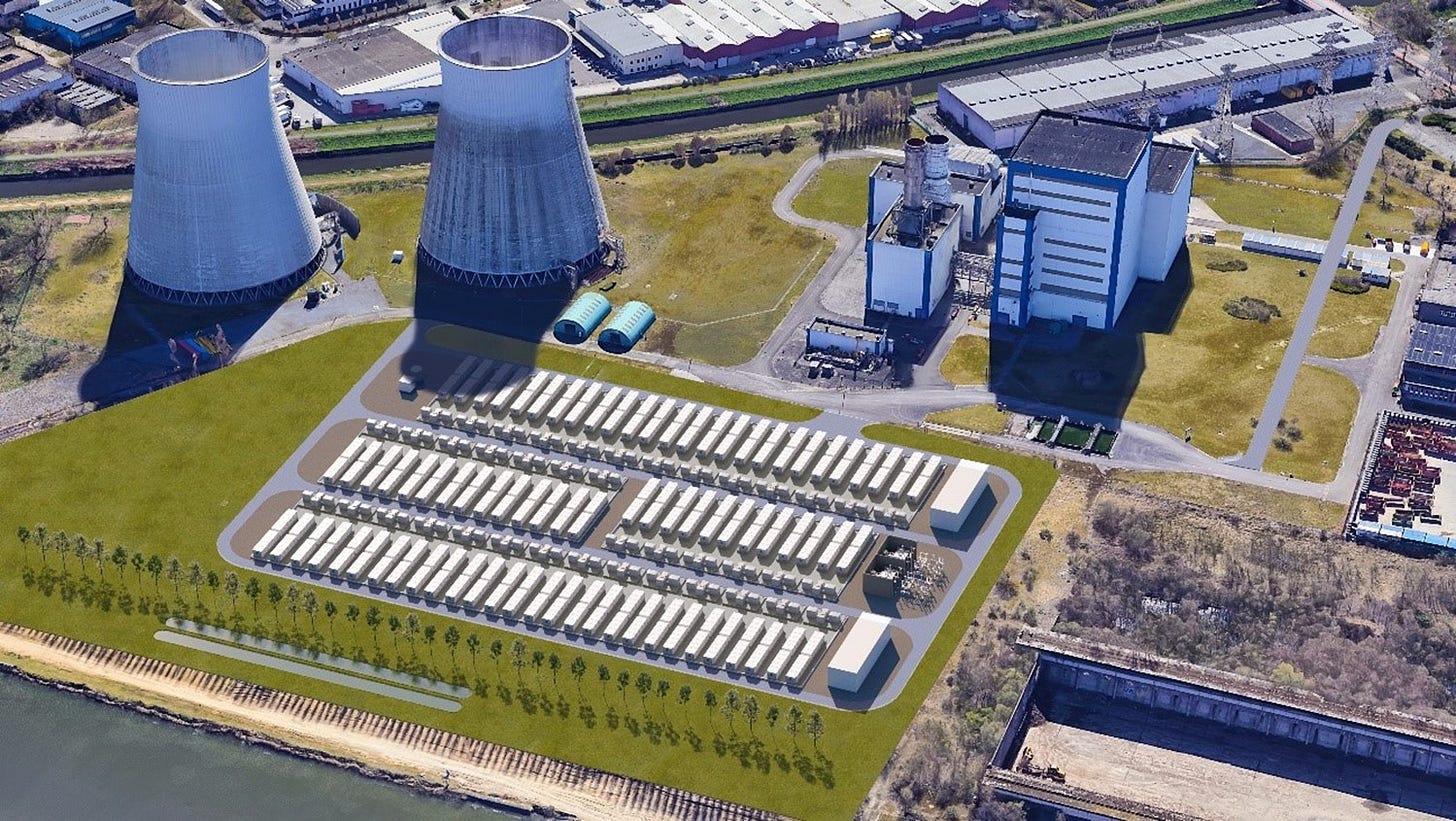
This modularity means that project implementation is relatively fast, and certainly much faster than most traditional large electrical projects such as transmission lines and thermal power plants.
The acceleration of large-scale projects
With decreasing costs and inherent modularity, it is not surprising to see an acceleration in the adoption of battery projects. Additionally, we are observing three concomitant elements happening simultaneously:
An increase in the number of projects and actors involved.
Higher installed power capacity, with current projects reaching up to 30 MW in the Netherlands or 50 MW in Belgium, and new planned projects expected to reach 200 MW or more.
Longer durations, with past projects typically lasting around one hour, while new projects are designed to last 2 to 4 hours, or even up to 8 hours. This extended duration is particularly evident in Germany, as shown in the graph below.
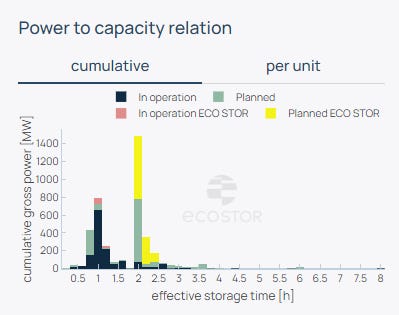
The main revenues: ancillary services and energy arbitrage
Of course, these projects are not being built without purpose. There are various revenue streams that large-scale batteries can capitalize on as described in this recent article.
There are four main revenue streams available for BESS investments – the primary frequency containment reserve (FCR), which TSOs use to correct short-term grid imbalances, secondary reserve markets, the arbitrage market and the capacity market, in which long-term contracts are signed to ensure that there is sufficient energy available if primary and secondary reserves are exhausted.
From my perspective, most revenue streams for large-scale batteries can be grouped into two main categories6:
Ancillary Services or Balancing: This includes services like FCR, aFRR, and even slower reserves such as mFRR. In some countries, such as the Netherlands and Belgium, passive balancing (also called reactive balancing), which involves reacting to the imbalance price, can also be classified as an ancillary service as it helps grid operators maintain balance7.
Energy Arbitrage: This involves activities in intraday and day-ahead markets, where batteries store electricity when it is cheap and sell it when prices are high. Additionally, colocating batteries with renewable assets or large loads can be considered part of energy arbitrage. In this setup, energy is stored for later use or sale when it is more economical, with the added benefit of potentially reducing grid fees (e.g., through peak shifting to lower peak power consumption).
Moreover, the energy markets for ancillary services are closely intertwined with energy markets, particularly continuous intraday markets. This connection is especially noticeable when there is a clear expectation of a large imbalance price, prompting market players to buy more aggressively in the continuous intraday markets to address potential imbalance positions8.
Another revenue: capacity payments
Furthermore, another potential revenue stream is the capacity market, also known as capacity payments, which goes by different names in different countries. Essentially, this market involves payments to assets simply for being available. In Belgium, the capacity market is referred to as the "Capacity Remuneration Mechanism" or CRM. This market will offer a fixed revenue stream, in addition to revenues from ancillary services and energy arbitrage, to approximately 1.1 GW of batteries in Belgium.
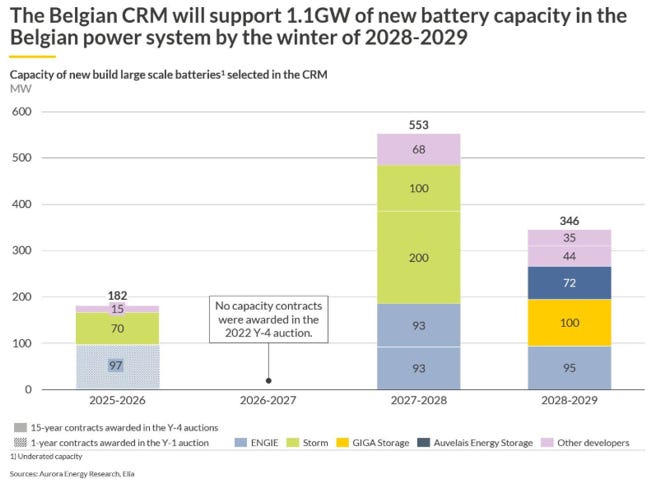
Various countries are setting such a capacity market for supporting the extension of large-scale batteries or in the reflection of doing doing such as Germany.
Towards the saturation of some market segments?
Interestingly, we can identify a certain pattern: batteries initially enter markets with the highest power-to-energy ratio, often the most profitable per MWh installed. As these markets become saturated, batteries move to the next ones in terms of power-to-energy ratio. In Europe, this means that FCR tends to get saturated first (which is already happening in some countries), followed by aFRR, along with the most lucrative short intraday opportunities and/or passive balancing. Below, we can see a similar trend unfolding in the UK.
In general, market depth is greater in markets with lower margins. For example, energy arbitrage in day-ahead markets is much larger than in continuous intraday trading, but the margins are lower. Therefore, batteries will initially reduce the margins in the markets with higher returns and gradually move to other markets.
Estimating the saturation levels for each market is challenging due to the influence of various factors9. Additionally, since large-scale batteries can provide all services across all timespans, they are likely to close any potential arbitrage opportunities between markets, leading to a harmonization of revenues. Currently, a battery operating in certain markets can earn varying revenues (see the example below). However, as more battery capacity becomes active across all markets, I expect these revenue differences to diminish.
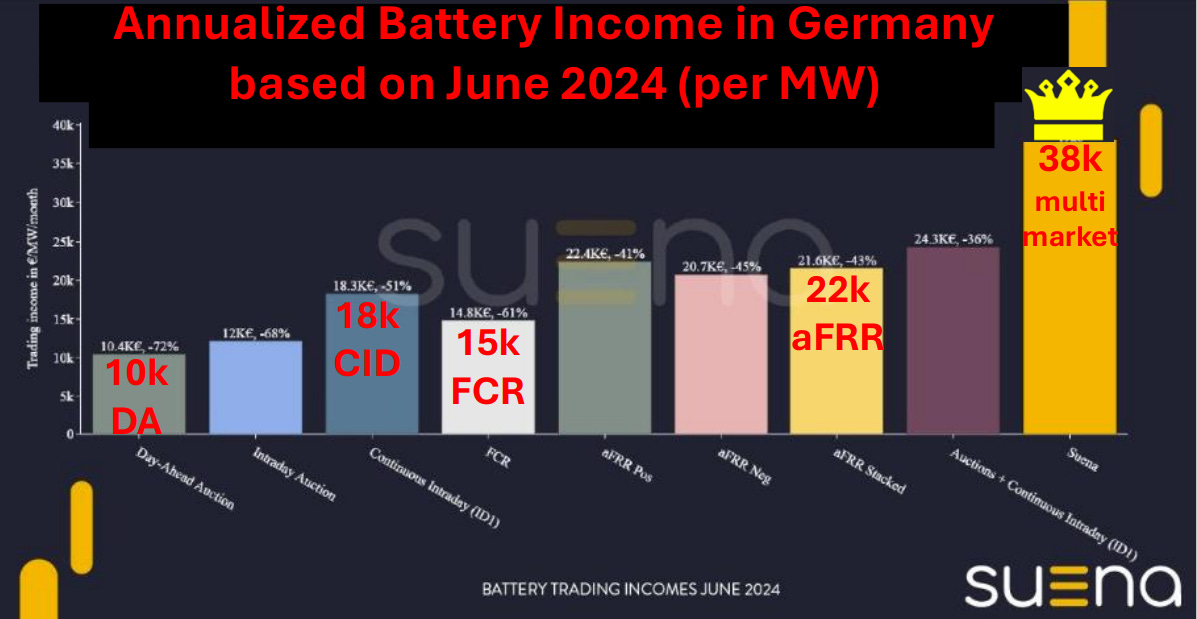
Large-scale or residential batteries?
There is significant hope that distributed batteries, particularly residential batteries, could become as important as large-scale batteries. Nevertheless, I believe this might not necessarily be the case10.
First, the cost difference between stand-alone residential systems and large-scale batteries is substantial. According to the graph below from Lazard, the "Levelized Cost of Storage" (LCOS) for unsubsidized residential systems ranges from $1157 to $1445 per kW per year, whereas utility-scale storage with the same duration has an LCOS of $214 to $374 per kW per year.
Furthermore, as explained by Lion Hirth11 home batteries might provide negligible benefits to the grid operations as the financial incentives of the battery owner is generally not well aligned with the overal system benefits. From his post (translated automatically from German):
Storage systems pay off financially because they allow you to consume more self-generated electricity. This is attractive because it saves grid fees, taxes and levies. But: of course, that doesn't do anything for society. Self-consumption is basically a tax-saving model.
Unfortunately, storage systems are then of no use for the network or system. They simply charge fully as soon as the sun rises. And at some point they are full. Then the solar system feeds into the grid. The stupid thing is: this is often around lunchtime, when the market and grid are overflowing with electricity anyway. From the point of view of the electricity system, this is even worse than no battery at all.
Consequently, I would argue that home batteries should not be prioritized, even with support schemes and tax incentives. They are significantly more expensive than large-scale projects, and current incentives are far from being aligned to make them beneficial from a global system perspective.
Fundamentally changing how the balance is maintained?
A final consideration is the potential change that we might have to introduce with the introduction of GWs of large-scale batteries. Likely, they will become the primary providers of all power reserves in the coming years.
Power reserves have been designed with a different activation period and timeframe. Traditionally, slower assets provide slower reserves. However, with large-scale batteries, the ramp rate—the speed at which an asset can change its power output—is extremely high compared to any other asset. Batteries can switch from maximal absorption to maximal injection within seconds.
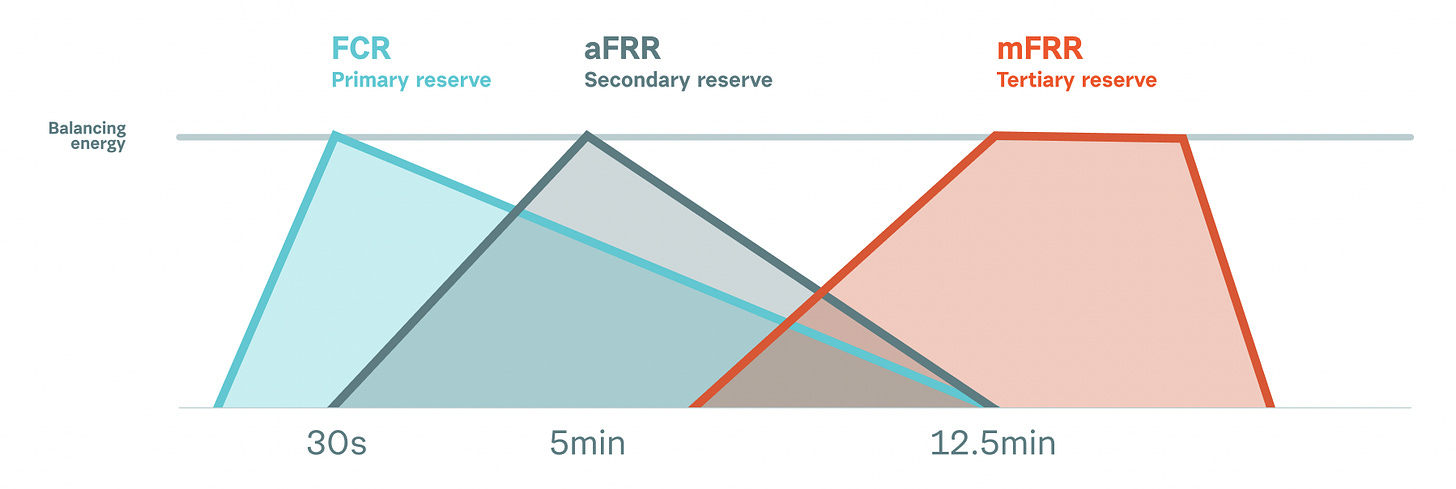
This ability to change the power output almost instantaneously could render the difference between fast and slow reserves less useful, in a grid where large-scale batteries dominate the provision of ancillary services. Lion Hirth12 is even going beyond by suggesting that (translated from German):
The electricity market currently operates in 15-minute cycles. This is far too slow for batteries because they switch so quickly. In the short term, we need ramp rules and in the long term, a reduction in the market time unit to 1 minute.
The logic is clear: our current market structure (15-minute cycles) and our organization of power reserves were designed when this technology was completely absent. With the rapid rise of large-scale batteries, we may need to rethink how we maintain grid balance.
In a nutshell
Let’s summarize in a few bullet points:
Lower costs and project modularity (along with associated benefits) are driving the market for large-scale batteries.
The growth has been astounding, with the installed energy storage capacity tripling in 2024 compared to 2023, and this trend appears set to continue.
The primary revenue streams are from ancillary services (balancing) and energy arbitrage, with capacity payments serving as a third avenue.
It is difficult to predict when a specific market will become saturated with batteries, but in the long run, I expect battery margins in each market to be similar or close to each other.
Large-scale batteries are likely to have such a significant impact that a consequential redesign of our grid operations and markets might be necessary. However, the adoption of large-scale batteries will likely progress faster than any of such consequential reforms.
This is my final post for the year 2024. I hope you have enjoyed reading them. Wishing you all a wonderful New Year, and see you in 2025!
Julien
BESS stands for battery energy storage system.
From their website: To bring it to fruition, the Group is investing between 230 and 290 million euros.
A gas peaker plant: a gas power plant that is used with very low utilization rates, only in times of scarcity.
This is certainly true for providing power reserves. For providing energy at a longer timescale, such as during days with low wind and solar, gas power plants are likely to be still useful. The proportions of both are difficult to foresee as it depends on the relative costs of both technologies.
We can also have more exotic revenues such as “grid investment deferrals”, which are not yet well organized and formalized. This refers to the postponement of grid investment via batteries.
One could also argue that this is more energy arbitrage as the battery would react to a price signal.
Such as the capacity mix, the situation in neighboring countries, the availability of other flexibility means, etc.
Statistics for 2024 have shown that, as the capacity of large-scale batteries has increased threefold while the capacity in the residential segment has decreased.
Batteries are a very expensive way to make a disfunctional power system slightly less disfunctional.
I like the information dissemination here.
It is important to note that battery devices have a very low energy density. Lithium is about 0.1 MJ/Kg. Diesel is about 43. Nuclear is in the millions.
The Law's of Physics and Thermodynamics still hold true, Batteries cannot deliver the energy needed even for widespread back up use. Good for cell phones and electronics though.